Time Travel Research Center
© 2005 Cetin BAL - GSM:+90 05366063183 - Turkey / Denizli
Combined Fission/Fusion Weapons
All nuclear weapons that are not pure fission weapons use fusion reactions to
enhance their destructive effects. All weapons that use fusion require a fission
bomb to provide the energy to initiate the fusion reactions. This does not
necessarily mean that fusion generates a significant amount of the explosive
energy, or that explosive force is even the desired effect.
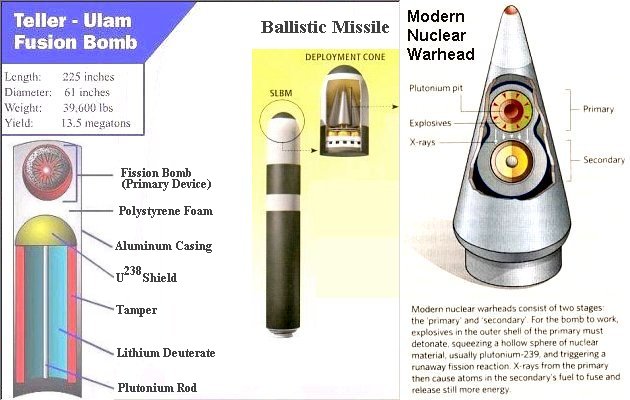
Fusion bombs, also called thermonuclear bombs, have higher kiloton yields and
greater efficiencies than fission bombs. To design a fusion bomb, some problems
have to be solved:
- Deuterium and tritium, the fuel for fusion, are both gases, which are hard
to store.
- Tritium is in short supply and has a short half-life so the fuel in the
bomb would have to be continuously replenished.
- Deuterium or tritium have to be highly compressed at high temperature to
initiate the fusion reaction
Boosted Fission Weapons
The first boosted fission weapon test was Greenhouse Item (45.5 kt, 24 May
1951), an oralloy design exploded on island Janet at Enewetak. The design of
the boosted fission weapon is a bit more complex than the pure fission weapons.
Contained in these weapons is a deuterium and tritium gas mixture, isotopes
of hydrogen. Tritium is a very expensive material to make, and it decays
at a rate of 5.5% per year, but the small amounts required for boosting (a few
grams) make its use economical. The deuterium/tritium gas mixture is included
in the center of the fissile material. When the bomb core undergoes enough
fission, it becomes hot enough to ignite the D-T fusion reaction which proceeds
swiftly. The reaction of the D-T fusion produces an intense burst of high-energy
neutrons that in turns causes a correspondingly intense burst of fissions in the
core. This greatly accelerates the fission rate in the core, thus allowing a
much higher percentage of the material in the core to fission before it blows
apart. Typically no more than about 20% of the material in an average size pure
fission bomb will split before the reaction ends (it can be much lower, the
Hiroshima bomb was 1.4% efficient). By accelerating the fission process a
boosted fission bomb increase the yield 100% (an unboosted 20 kt bomb can thus
become a 40 kt bomb). The actual amount of energy released by the fusion
reaction is negligible, about 1% of the bomb's yield, making boosted bomb tests
difficult to distinguish from pure fission tests (detecting traces of tritium is
about the only way).
Staged Radiation Implosion Weapons
Although boosting can multiply the yield of fission bombs, it still has
the same fundamental fission bomb design problems for high yield designs. The
boosting technique is most valuable in small light-weight bombs that would
otherwise have low efficiency. Soon later, this lead to the Staged Radiation
Implosion Weapons which is more commonly know as the Teller-Ulam weapon or (depending
on type) fission-fusion or fission-fusion-fission weapons. These class of
weapons incorporate light elements such as hydrogen and lithium to overcome the
limitation yield of the fission type device and, as well, the boosted fission
weapons designs. Other reason for the new design of the Teller-Ulam device is
the reduction of weapon cost by reducing the amount of costly enriched uranium-235
or plutonium-239. The new design, Teller-Ulam device, also allows for lighter
weight than previous designs. Obvious advantages includes: transportation (smaller
aircraft can be used to deploy weapon) and rocket size and fuel to deploy is
reduced.
It is inconvenient to carry deuterium and tritium as gases in a thermonuclear
weapon, and certainly impractical to carry them as liquefied gases, which
requires high pressures and cryogenic temperatures. Instead, one can make a
“dry” device in which 6Li is combined with deuterium to form the
compound 6Li D (lithium-6 deuteride). Neutrons from a fission
“primary” device bombard the 6 Li in the compound, liberating tritium,
which quickly fuses with the nearby deuterium. The alpha particles, being
electrically charged and at high temperatures, contribute directly to forming
the nuclear fireball. The neutrons can bombard additional 6Li nuclei
or cause the remaining uranium and plutonium in the weapon to undergo fission.
This two-stage thermonuclear weapon has explosive yields far greater than can be
achieved with one point safe designs of pure fission weapons. Thermonuclear
fusion stages can be ignited in sequence to deliver any desired yield. Such
bombs, in theory, can be designed with arbitrarily large yields: the Soviet
Union once tested a device with a yield of about 59 megatons.
In a relatively crude sense, 6 Li can be thought of as consisting of an alpha
particle ( 4He) and a deuteron ( 2H) bound together. When
bombarded by neutrons, 6Li disintegrates into a triton ( 3H)
and an alpha: 6 Li + Neutron = 3H + 3He + Energy
The nuclear fusion reaction which ignites most readily is: D + T = 4He
+ n + 17.6 MeV.
The fusion reactions are used to boost the yield in two ways:
- By directly releasing a large amount of energy in fusion reactions;
- By using high-energy or "fast" neutrons generated by fusion to release
energy through fissioning of a fissionable jacket around the fusion stage. In
the past this jacket was often made of natural or depleted uranium, so that
energy is produced by fast fission of cheap U-238. Thorium may also be used
for this purpose, although it is inferior to cheap and abundant depleted
uranium. Weapons in which there is a premium on weight and size (i.e.
virtually all modern strategic weapons) use moderately to highly enriched
uranium as the jacketing material.
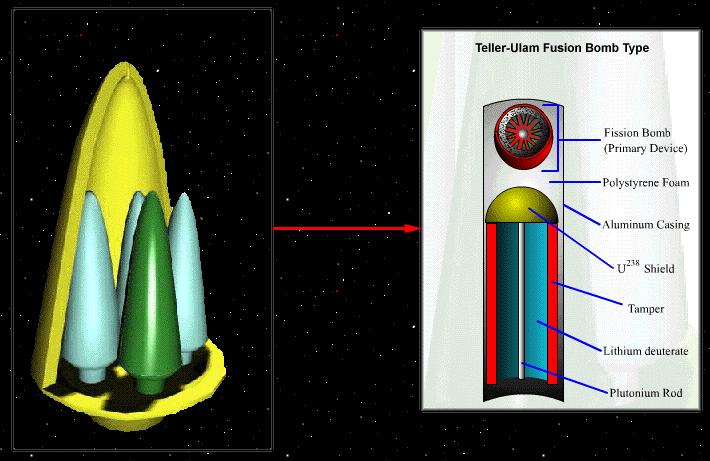
In other words, the Teller-Ulam configuration makes use of the
fact that at high temperatures of a fission bomb about 80% or more of the energy
exists as soft X-rays, not as kinetic energy (heat). The rate at which the
energy from radiation expands from the core is about 1000km/sec faster than the
heat. The energy that is released from the core as heat is by far slower than
the radiation (X-rays). This, of course is taken advantage, in the Teller-Ulam
design. These dynamics of the Teller-Ulam design make it possible to compress,
and ignite a physically separate mass of fusion fuel (the second stage) through
radiation implosion (X-rays) before the expanding trigger disrupts it.
Neutron Bombs
Neutron bombs, more formally referred to as "enhanced radiation
(ER) warheads", are small thermonuclear weapons in which the burst of neutrons
generated by the fusion reaction is intentionally not absorbed inside the weapon,
but allowed to escape. This intense burst of high-energy neutrons is the
principle destructive mechanism. Neutrons are more penetrating than other types
of radiation so many shielding materials that work well against gamma rays do
not work nearly as well. The term "enhanced radiation" refers only to the burst
of ionizing radiation released at the moment of detonation, not to any
enhancement of residual radiation in fallout.
The U.S. has developed neutron bombs for use as strategic anti-missile
weapons, and as tactical weapons intended for use against armored forces. As an
anti-missile weapon ER weapons were developed to protect U.S. ICBM silos from
incoming Soviet warheads by damaging the nuclear components of the incoming
warhead with the intense neutron flux. Tactical neutron bombs are primarily
intended to kill soldiers who are protected by armor. Armored vehicles are
extremely resistant to blast and heat produced by nuclear weapons, so the
effective range of a nuclear weapon against tanks is determined by the lethal
range of the radiation, although this is also reduced by the armor. By emitting
large amounts of lethal radiation of the most penetrating kind, ER warheads
maximize the lethal range of a given yield of nuclear warhead against armored
targets.
One problem with using radiation as a tactical anti-personnel
weapon is that to bring about rapid incapacitation of the target, a radiation
dose that is many times the lethal level must be administered. A radiation dose
of 600 rads is normally considered lethal (it will kill at least half of those
who are exposed to it), but no effect is noticeable for several hours. Neutron
bombs were intended to deliver a dose of 8000 rads to produce immediate and
permanent incapacitation.
The neutron flux can induce significant amounts of short lived
secondary radioactivity in the environment in the high flux region near the
burst point. The alloy steels used in armor can develop radioactivity that is
dangerous for 24-48 hours. If a tank exposed to a 1 kt neutron bomb at 690 m (the
effective range for immediate crew incapacitation) is immediately occupied by a
new crew, they will receive a lethal dose of radiation within 24 hours.
Due to the rapid attenuation of neutron energy by the atmosphere (it drops by
a factor of 10 every 500 m in addition to the effects of spreading) ER weapons
are only effective at short ranges, and thus are found in relatively low yields.
ER warheads are also designed to minimize the amount of fission energy and blast
effect produced relative to the neutron yield. The principal reason for this was
to allow their use close to friendly forces. The common perception of the
neutron bomb as a "landlord bomb" that would kill people but leave buildings
undamaged is greatly overstated. At the intended effective combat range (690 m)
the blast from a 1 kt neutron bomb will destroy or damage to the point of
unusability almost any civilian building. Thus the use of neutron bombs to stop
an enemy attack, which requires exploding large numbers of them to blanket the
enemy forces, would also destroy all buildings in the area.
Neutron bombs (the tactical versions at least) differ from other
thermonuclear weapons in that a deuterium-tritium gas mixture is the only fusion
fuel. The reasons are two-fold: the D-T thermonuclear reaction releases 80% of
its energy as neutron kinetic energy, and it is also the easiest of all fusion
reactions to ignite. This means that only 20% of the fusion energy is available
for blast and thermal radiation production, that the neutron flux produced
consists of extremely penetrating 14.7 Mev neutrons, and that a very small
fission explosion (250-400 tons) can be used for igniting the reaction. The more
typical lithium deuteride fuel would produce much more blast and flash for each
unit of neutron flux, and would require a much larger fission explosion to set
it off. The disadvantage of using D-T fuel is that tritium is very expensive,
and decays at a rate of 5.5% a year. Combined with its increased complexity this
makes ER warheads more expensive to build and maintain than other tactical
nuclear weapons. To produce a 1 kt fusion yield 12.5 g of tritium and 5 g of
deuterium are required.
Radiological Weapons ("Salted" or Doomsday
Device)
A "salted" nuclear weapon is reminiscent of fission-fusion-fission weapons,
but instead of a fissionable jacket around the secondary stage fusion fuel, a
non-fissionable blanket of a specially chosen salting isotope is used (cobalt-59
in the case of the cobalt bomb). This blanket captures the escaping fusion
neutrons to breed a radioactive isotope that maximizes the fallout hazard from
the weapon rather than generating additional explosive force (and dangerous
fission fallout) from fast fission of U-238.
Variable fallout effects can be obtained by using different salting isotopes.
Gold has been proposed for short-term fallout (days), tantalum and zinc for
fallout of intermediate duration (months), and cobalt for long term
contamination (years). To be useful for salting, the parent isotopes must be
abundant in the natural element, and the neutron-bred radioactive product must
be a strong emitter of penetrating gamma rays.
Candidate Salting Agents |
Parent Isotope |
Natural abundance |
Radioactive Product |
Half-Life |
Cobalt-59 |
100% |
Co-60 |
5.26 years |
Gold-197 |
100% |
Au-198 |
2.697years |
Tantalum-181 |
99.99% |
Ta-182 |
115days |
Zinc-64 |
48.89% |
Zn-65 |
244days |
The idea of the cobalt bomb originated with Leo Szilard who publicized it in
Feb. 1950, not as a serious proposal for weapon, but to point out that it would
soon be possible in principle to build a weapon that could kill everybody on
earth. To design such a theoretical weapon a radioactive isotope is needed that
can be dispersed world wide before it decays. Such dispersal takes many months
to a few years so the half-life of Co-60 is ideal.
The Co-60 fallout hazard is greater than the fission products from a U-238
blanket because
- many fission-produced isotopes have half-lives that are very short, and
thus decay before the fallout settles or can be protected against by short-term
sheltering;
- many fission-produced isotopes have very long half-lives and thus do not
produce very intense radiation;
- the fission products are not radioactive at all.
The half-life of Co-60 on the other hand is long enough to settle out before
significant decay has occurred, and to make it impractical to wait out in
shelters, yet is short enough that intense radiation is produced.
Initially gamma radiation fission products from an equivalent size fission-fusion-fission
bomb are much more intense than Co-60: 15,000 times more intense at 1 hour; 35
times more intense at 1 week; 5 times more intense at 1 month; and about equal
at 6 months. Thereafter fission drops off rapidly so that Co-60 fallout is 8
times more intense than fission at 1 year and 150 times more intense at 5 years.
The very long lived isotopes produced by fission would overtake the again Co-60
after about 75 years.
Zinc has been proposed as an alternate candidate for the "doomsday role". The
advantage of Zn-64 is that its faster decay leads to greater initial intensity.
Disadvantages are that since it makes up only half of natural zinc, it must
either be isotopically enriched or the yield will be cut in half; that it is a
weaker gamma emitter than Co-60, putting out only one-fourth as many gammas for
the same molar quantity; and that substantially amounts will decay during the
world-wide dispersal process. Assuming pure Zn-64 is used, the radiation
intensity of Zn-65 would initially be twice as much as Co-60. This would decline
to being equal in 8 months, in 5 years Co-60 would be 110 times as intense.
Militarily useful radiological weapons would use local (as opposed to world-wide)
contamination, and high initial intensities for rapid effects. Prolonged
contamination is also undesirable. In this light Zn-64 is possibly better suited
to military applications than cobalt, but probably inferior to tantalum or gold.
As noted above ordinary "dirty" fusion-fission bombs have very high initial
radiation intensities and must also be considered radiological weapons.
No cobalt or other salted bomb has ever been atmospherically tested, and as
far as is publicly known none have ever been built. In light of the ready
availability of fission-fusion-fission bombs, it is unlikely any special-purpose
fallout contamination weapon will ever be developed.
Fission Weapons
These are weapons that only use fission reactions as a source of energy.
Fission bombs operate by rapidly assembling a sub critical configuration of
fissile material (plutonium or enriched uranium) into one that is highly
supercritical. The original atomic bombs tested in 16 July 1945 (device name:
Gadget, test name: Trinity) and dropped on Japan in 6 August 1945 (Little Boy,
over Hiroshima) and 9 August 1945 (Fat Man, over Nagasaki) were pure fission
weapons.
A fission weapon utilizes Plutonium-239 or Uranium-235 to create a high
energy explosion. U-235 and Pu-239 are the only two elements that are used in
fission type nuclear weapons. U-235 and Pu-239 hold unique properties in which
when a neutron collides with the nucleus of the element the U-235 or Po-239 will
split into two halves, releasing neutrons. The U-235 releases 2-3 neutrons per
fission causing other fissions. However, Pu-239 is preferred over U-235. Pu-239
has higher fission and scattering cross sections than U-235, and a larger number
of neutrons produced per fission, and consequently a smaller critical mass is
needed. The amount of neutron release is dependent on how the atom splits. The
two new atoms that are formed release gamma radiation while settling into their
new states.
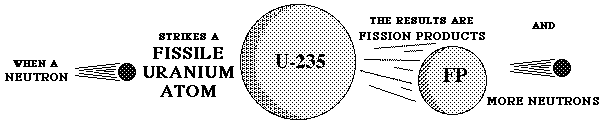
The condition known as super-criticality is referring to the
high probability of a U-235 or Pu-239 capturing a neutron. In a bomb working
correctly, more than one neutron ejected from each fission causes another
fission to occur. The process of capturing the neutron and splitting happens
very quickly, on the order of picoseconds (1*10E-12 seconds). Enormous amounts
of energy is released on the occurrence of a fission and can be calculated.
Multiples effects occur when the atom splits: new elements are formed, neutrons
are released, and gamma radiation is emitted. However, if the masses of the new
elements formed are added to the neutrons emitted, it weighs less than the
original element (U-235 or Pu-239). The difference in weight is converted into
energy with Einstein's formula E=mc^2 (where: E=energy, m=mass (kg), c=speed of
light (3.0x10^8m/s) ). To put in perspective a pound of highly enriched uranium
is equal to on the order of a million gallons of gasoline. A pound of U-235 is
smaller than a baseball, while a million gallons of gasoline would fill a
building with dimension 50 x 50 x 50feet.
In order for a sustained nuclear reaction enriched U-235 must be used. The
majority of Uranium in the world is U-238 only a small percentage of U -235
exists. Special facilities such as the Isotope separation production facilities
at Oak Ridge during WWII can yield enriched uranium. Furthermore, the fuel in a
fission bomb must be kept separate (sub-critical) to prevent premature
detonation. Only at detonation do the masses come together to ensure critical
mass (critical mass - is the minimum mass of fissionable material required to
sustain a nuclear fission reaction). This procedure of keeping the masses
separate (sub-critical) to prevent detonation bring about problems in design.
To sustain a fission reaction the sub-critical masses must be brought together,
which will provide adequate supply of neutrons to ensure a fission reaction.
Neutrons must be introduced into the critical-mass to let fission begin.
Perhaps the most concern is with fizzle. A reaction starts, but not fast enough
and the bomb fizzles out. So when detonated, as much of the material as
possible must be fissioned before the bomb explodes.
These problems are solved by two processes; the gun-triggered device or the
implosion device. Neutrons are introduced to the supercritical mass by a
neutron generator. The neutron generator consists of a small pellet of polonium
and beryllium. The Neutron generator is separated from the supercritical mass
by a foil. When detonation is needed the two sub critical masses are brought
together by either the gun-triggered device or the implosion device and the foil
is breached. Immediately the polonium begins to emit alpha particles. The
alpha particles, in turn, collide with the beryllium-9 to produce beryllium-8
and emit neutrons. The neutrons then collide into the core of the supercritical
masses beginning the chain reaction.
The core of the supercritical mass is designated to a tamper during fission.
This tamper is made of U-238. As the reaction intensifies the tamper is heated
and is expanding exerting pressure back onto the super critical core.
Furthermore, the tamper slows down the cores expansion ensuring as much as a
complete detonation of material as possible. Another purpose the tamper serves
is a neutron reflector. Neutrons from the reaction reflect neutron back to the
core providing a more efficient reaction.
Hiçbir
yazı/ resim izinsiz olarak kullanılamaz!! Telif hakları uyarınca
bu bir suçtur..! Tüm hakları Çetin BAL' a aittir. Kaynak gösterilmek şartıyla siteden
alıntı yapılabilir.
The Time Machine Project © 2005 Cetin BAL - GSM:+90 05366063183 -Turkiye/Denizli
Ana Sayfa /index /Roket bilimi /
E-Mail /CetinBAL/Quantum Teleportation-2
Time Travel Technology /Ziyaretçi
Defteri /UFO Technology/Duyuru
Kuantum Teleportation /Kuantum Fizigi
/Uçaklar(Aeroplane)
New World Order(Macro Philosophy)
/Astronomy
|